Are We Receiving
Biological Signals
from Outer Space?
By L. George Lawrence
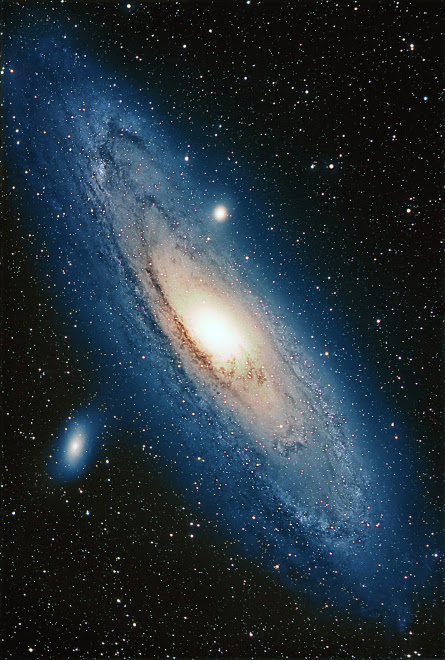
M31, Andromeda Galaxy.
Is there intelligent life elsewhere in the universe, and if so, what form does it take? Some new evidence points to some suprising, yet familiar, answers.
There here have been many hints that a dominant biological force is responsible for our creation. To lend credence to that theory we now have some evidence, provided by the techniques of remote astrobiological-image sensing (RAIS for short), that the human form or "likeness" may indeed be typical of all intelligent life in space. Before we discuss the evidence, we should explain the technology involved.
Not to be confused with radio astronomy, RAIS is made possible with "biosensors." The data they produce is called a "signature plate" or "biogram." These deep-space scans are conducted from uninhabited desert regions at night to safeguard the equipment against electro-magnetic interference. Encompassing 18 years of research, this article will profile the general aspects of RAIS and show the evolution of its equipment. Literature and patent references are provided in a boxed text for experimenters desiring to make their own contributions to this promising field.
Biosensors. Biosensors, also known as biological transducers, emerged from general research in chemistry, biology, and electronics. Basic sensors are comprised of biologically sensitive materials such as an enzyme, antibody, organelle, bacterial or other cell, sections of plant or animal tissue, or proprietary substrates capable of converting a bio-chemical signal into an electrical signal. Such a material can be sensitized to render an electronic signal in the presence of external fields and forces. The signal(s) can be detected, taped, and further processed by conventional means.
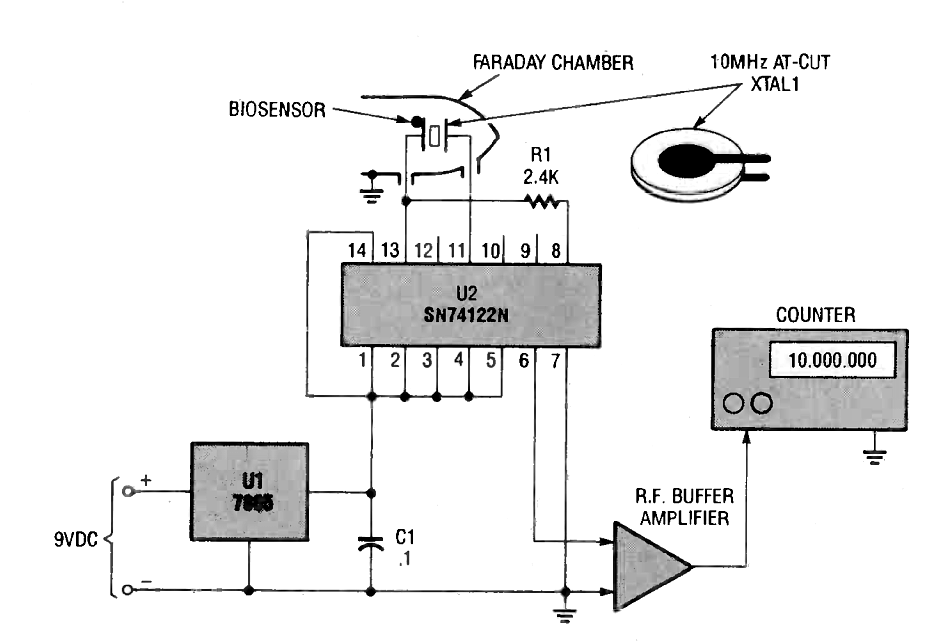
Fig. 1. This is a quartz-crystal biosensor assembly complete with oscillator and AT-cut quartz plate. Changes in the biosensor affect the operating frequency and thus the counter readout.
One of the easiest biosensors to make is the quartz type, as shown in Fig. 1. There, a 10-MHz AT-cut crystal is used in an oscillator circuit driven by U2, a SN74122N. The crystal itself is housed in a protective Faraday chamber and the organic substance is bonded to one side of the crystal plate. If the sensory substance is exposed to, say, a gas capable of acting on it, the absorption of the gas will cause a change in the oscillator's frequency. Sensors of this type are able to detect gas at ultra-low (part-per-billion or part-per-trillion) concentrations, an ability which equals the sensitivity of a dog's nose.
Aside from astronomical applications, the quartz-crystal technique has proven exceptionally useful for an assay of substrates: formaldehyde in the air can be detected with formaldehyde dehydrogenase, contraband drugs can be detected with cocaine or morphine antibodies, etc. In all of these instances, the key for inducing changes in crystals is the use of highly reactive organic materials. The crystal plates must be washed prior to use. See the patents mentioned in the boxed text for information on sample preparation.
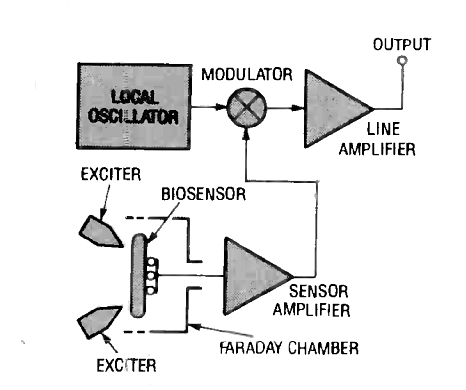
Fig. 2. A cell-type biosensor assembly beats the biological signals with a local carrier oscillator via a modulator.
Living biosensors containing active cells have special properties. The membranes of most cells act as insulators at low electrical frequencies. Currents will flow around them, but not through them. Between 1 kHz and 100 kHz, the impedance of a single suspension of cells drops quite sharply to a lower value. The impedance of the living cytoplasm may then be represented as a resistivity, expressed in ohms/cm.
The most impressive aspect of cell resistivity is the similarity from one cell type to another. Egg cells, plant cells, animal cells, and nerve axons all overlap in their electrical constants. It is only by means of judicious catalyzation and excitation that a particular function can be established for a sensor. Figure 2 shows a basic arrangement for image acquisition. The biosensor is placed in a shielding Faraday chamber. Any arising signals are amplified and fed into the modulator. Functioning in an AM mode to secure strong carrier modulation, the signal then enters the line amplifier for final processing and transcription. The photos show the actual field instruments. Figure 3 depicts the signal-processing operations required. The building blocks shown can be arranged and combined in various degrees of sophistication.
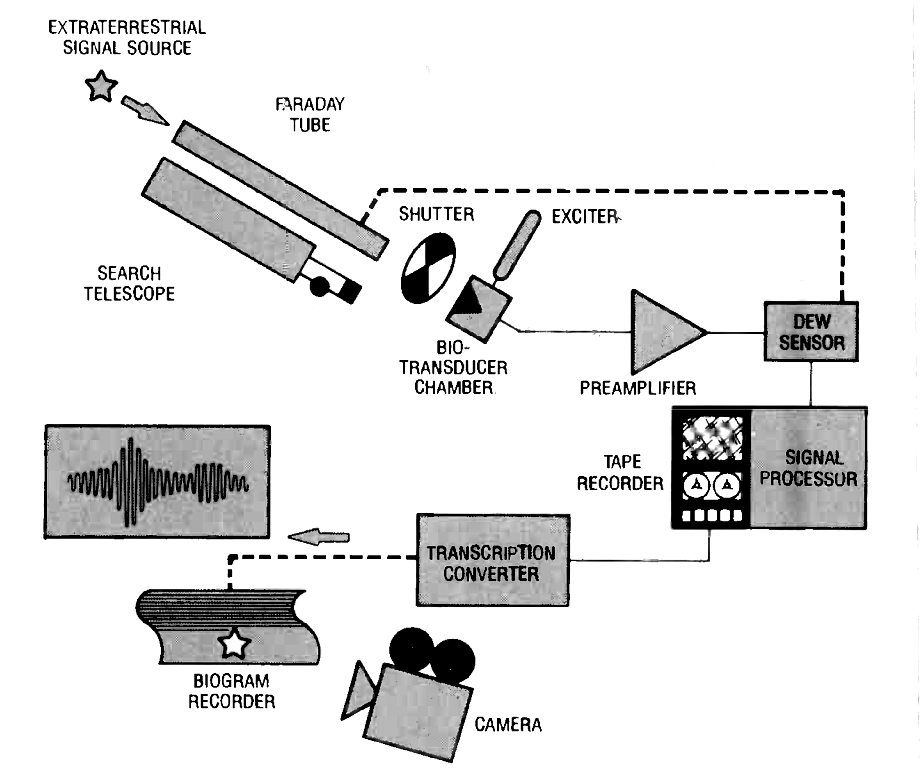
Fig. 3. This represents the data flow of a biogram field unit. The Biogram is recorded in helical-scan mode.
Background. The initial discovery of biological signals of galactic origin dates back to 1971. Accidental and unexpected, the discovery transpired during ecological remote-sensing research at Oak Grove Park, California, then using experimental organic semi-conductors as primary-event transducers. First apparent as a strange sequence of audio modulations, which were recorded on tape, the signals or induced continuum modulations (IOM's) reached the ecological field station from astronomical coordinates 10h 40m right ascension, + 56° north declination. It placed the source in the Ursa Major sector and eventually directed attention to a large number of galaxies in the vicinity of Phecda, one of the Big Dipper's most prominent stars. Over a period of years, investigations shifted toward reasonably close galaxies such as Andromeda and its companions, the elliptical galaxies M32 and NGC 205. The local Milky Way system was explored as well.
It was during these decade-long operations that the nocturnal data tapes began to reveal discrete intensity modulations, which implied the presence of coherent visual information. Most startling were the long time intervals of 2 minutes or more, plus the absence of sync information as we understand it. It would appear that an entire image was transmitted at once, similar to a picture produced by a film projector. Like a film projector's imagery, the pictures expand as they travel through space. The propagation width of the biological pictures are enormous — truly astronomical! These observations and others led to the conclusion that the source is at some cosmological distance from us, and that the images are not a purely natural phenomenon.
Astronomical Systems. Astronomy, as other disciplines, has its own vocabulary of words and phrases with precise technical definitions. However, its terminology is less complex than computer jargon. It is necessary to use a little astronomical vocabulary to describe the mechanical functioning of biogram apparatus. Don't let the new words throw you, we just want to give you a feeling of the large number of factors the designer of such machines must take into account.
Our galactic system is a member of many systems occupying an ellipsoidal volume of space. Our system is near one end, Messier 31 (the Andromeda galaxy) is at the other. Surveys by astronomers Shapley and Adams at Harvard Observatory of the 1,025 bright extra-galactic systems has made the distribution of these bodies apparent. To capture and lock onto a single target, it is necessary that telescope systems can automatically track the object and overcome the effects of the Earth's motion. A stationary, non-tracking telescope would only obtain circumpolar star trails — streaks in the film from the motion of the Earth. It is obvious that no viable galactic phenomena can be captured and recorded with such a device.
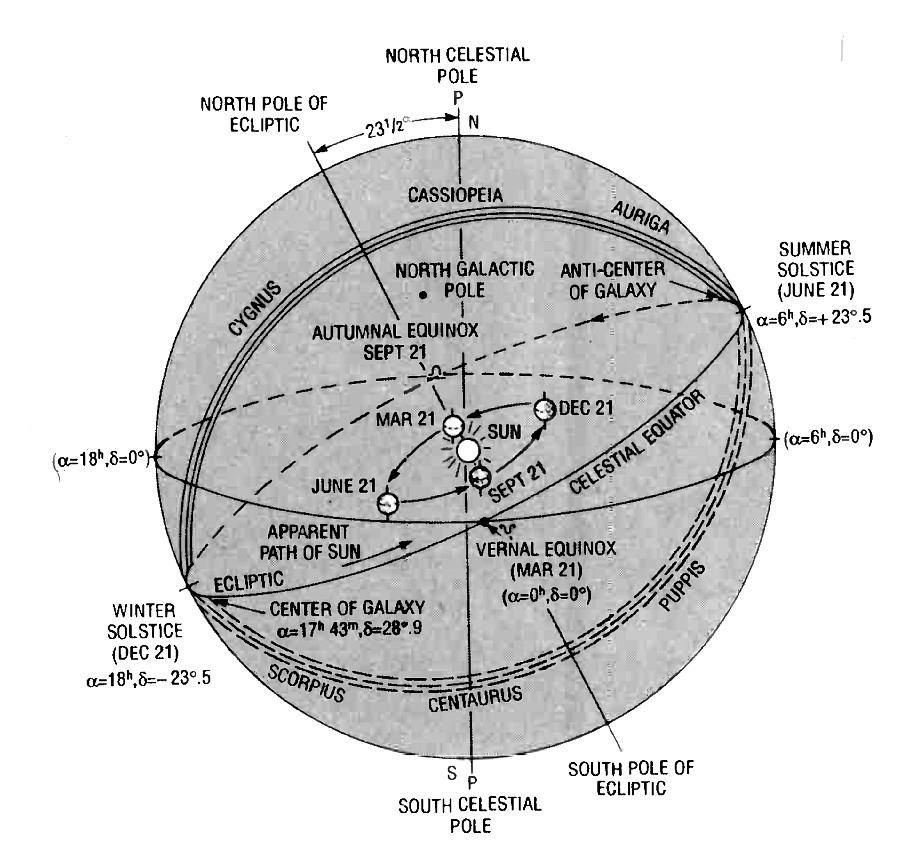
Fig. 4. All the aspects of the ecliptic system must be taken into account when tracking an astronomical body.
A tracking telescope must take into account right ascension and declination, the ecliptic, the galactic center, and the vernal and autumnal equinoxes for setting sidereal time (star time). Figure 4 shows some of these mandatory astronomical elements. Note that half of the celestial equator is above the horizon. Northward, the daily courses of the stars come up more and more over the horizon until they are entirely above it. Southward from the celestial equator, they are depressed more and more until they disappear completely. The vernal equinox (0h right ascension, 0° declination) is the point where the sun crosses the celestial equator on its way north, about March 21st. The autumnal equinox (12h right ascension, 0° declination) is the point where the sun crosses the celestial equator on its way south, about September 21st. The sideral day is about 3m 56s shorter than the solar day. The sidereal day ends when the Earth has made a complete rotation, bringing the vernal equinox again to upper transit. For those interested in astronomy, electronic clocks are available that produce a concise sidereal time-display.
Past and Current Systems. As was mentioned, a telescopic system must have the ability to track stars. Practically any solid, motor-driven mount suffices for astrobiological-image acquisition. However, large drives and mounts of the alt-azimuth type are required if the biosensor complex must be combined with a radiotelescope for detecting incident electromagnetic interference. Such a system was constructed in 1973 and operated for one year. The instrument confirmed the existence of biological signals as a new, non-electromagnetic reality. It also provided other insights which, in time, permitted the construction of a variety of portable field systems for desert use. The apparatus had a skirted radiotelescope with three biosensors, one of which is in the black QRB field holding the Faraday tube. A dust shroud kept the internal antenna and electronics clean. Pre-amplifiers, feeders, and auxiliaries were mounted on the telescope body.
One of the more recent equipment systems is a highly flexible search telescope adapted for biological sensing. The machine is largely all-electronic in terms of telescope operations, including tracking at both solar and sidereal rates of speed. The Faraday tube with its biological complex is mounted on the top. The motor-driven shutter aids in the detection of incident electromagnetic interference, which may cause sharp undulations in the biological signal train. The shutter eliminated the need for a heavy radiotelescope.
The unit was also equipped with tele-vision gear for pictorial extensions and video recording. It is hoped that, in time, moving renditions of biological images can be obtained. Much hinges on the quality of the biological substrates and on the ability of SuBICon's (summing biological-image converters) to operate under various forms of excitation using the traditional NTSC television format. Respective research is in progress.
It was by means of such and related equipment systems that the first few biograms could be recorded over a period of years. Image enhancement, has greatly assisted their evaluation.
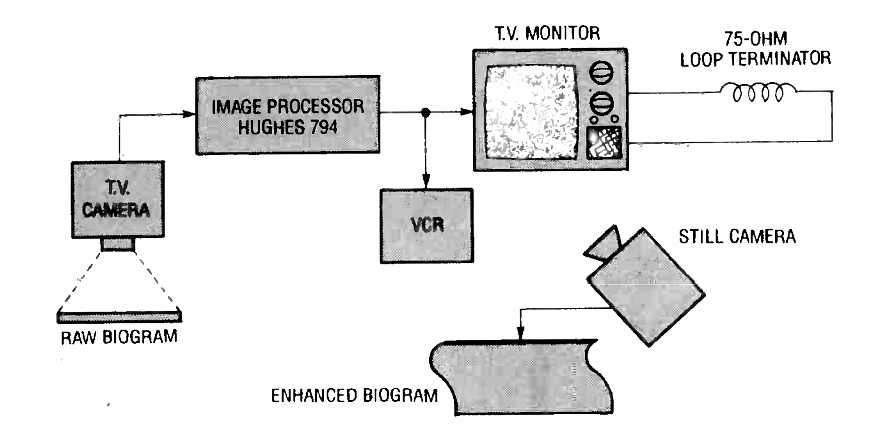
Fig. 5. This biogram image-enhancement system uses a Hughes Model 794 Processor to clarify incoming pictures.
Image Enhancement. Basically, image enhancement is a process whereby signature plates such as photographs and/or pictorial biograms are converted into digital information. The process provides a high degree of heretofore unobtainable resolution. Typically, a small area on a given plate is scanned with a microdensitometer and evaluated in terms of image density. The readings may be spaced only .015 to .05 millimeters apart. Each density number (DN) is then recorded as an 8- or 10-bit binary number. In the case of 8-bit data, for example, each DN records one of 256 levels of brightness numbered from 0 to 255, which is the value of the highest 8-bit binary number, 1111111. Pure black is equal to a DN of zero, while the highest DN (say. 255) is set at white. Gray tones result in intermediate DN's. That generates an immense amount of data. A 3cm2 portion of a plate may yield as much as 1,000,000 values. The digitized image is then reconstructed for video or photograhic recording, or viewing via a monitor. Figure 5 shows a block diagram of the standard processing steps.
The biograms shown in Figs. 6-9 were image-enhanced with the Hughes Model 794 Television Image Processor. The instrument's 16-bit memory provided up to 256 frames of image integration. The biogram's original grey level image was processed at 640 x 482 x 8 bits. Recursive filtering was used to improve signal-to-noise ratios.
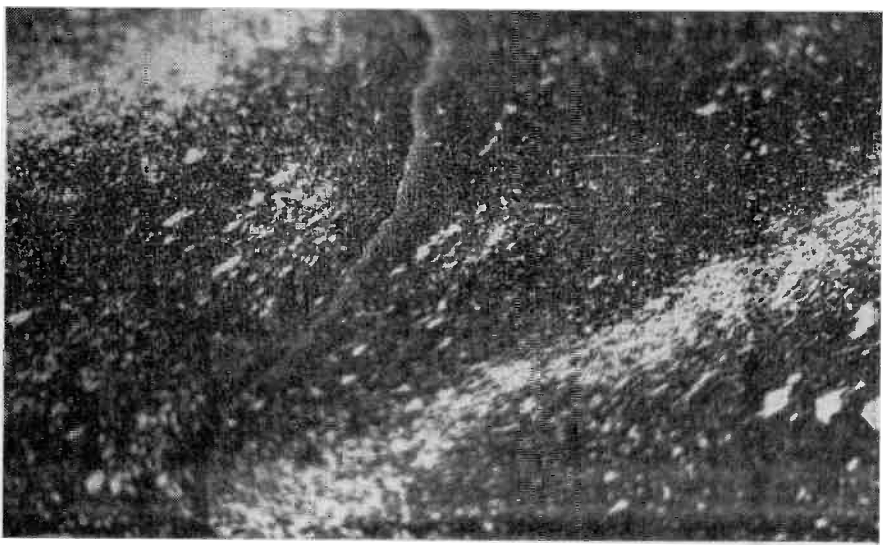
Fig. 6. The biogram shows the commencement of a biological image transmission. The image begins to form at upper left-hand side of the frame.
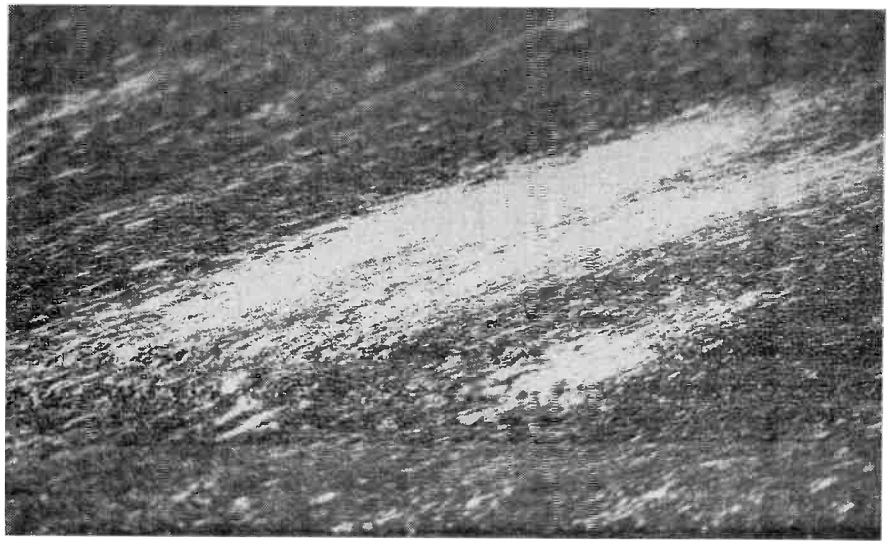
Fig. 7. The biogram shows a narrowing and intensification of the image with a satellite image begins to emerge.
The photos show the complete interception of a biogram from beginning through final image enhancement as follows: After having recorded countless blanks during thousands of desert nights, an image begins to form on the left-hand side of the frame (Fig. 6). Its enormous size is startling. Figure 7 shows a large white form with a smalller satellite image emerging (20 minutes have passed at this point). In Fig. 8 the large white image and satellite image have resolved into distinct, coherent forms. The data for the right-hand section of Fig. 8 was subjected to integration, continuous image-frame subtraction, and grey-level enhancement. Figure 9 shows the enhanced biogram's final appearance. That the biogram would indicate a glorious relief was totally unexpected. And so human an arm and form, yet big! It gave rise to cosmological reviews.
Biological Cosmology. In an important field such as this, it goes without gainsaying that no progressive course can be charted without a map. That is an understanding of the dynamics of biological cosmology. We need to determine the fundamental principles that define how life emerges and the shape it takes. Such studies are not an esoteric exercise, but vital to instrumentation development. Historically, physics and chemistry have come to an almost complete union in their understanding of the structure of matter. By contrast, biology deals with structures of an infinitely more complicated type. Thus the laws that govern their design are more complex.
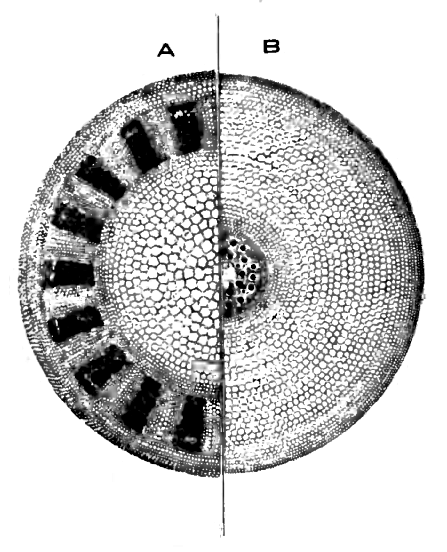
Fig. 10. These experimental biosensor substrates are taken from the root of a Jerusalem artichoke (A), and the root of an asparagus plant (B).
Take, for example, an experimental biosensor substrate shown in Fig. 10. Section A is the root of a Jerusalem artichoke; section B is the root of asparagus. Both items have a profound similarity, but that is due to their function rather than some universal law. Obviously the forces at play here must be handled with greater caution than processes of mechanical physics, chemistry, or electronics.
Nobel laureate Niels Bohr described this situation by saying that in biology, one is concerned with manifestations of possibilities. But where are the universal constants — the characteristic quantities — to support said possibilities? Universal constants determine the scale of nature. The velocity of light is one constant, Plank's constant is another. But judging from the immensity of our biograms, there must exist a third constant for purely dimensional purposes.
While searching for constants, some researchers have tried to set forth new cosmological principles involving the phenomenon of time, which is observed but not fully understood. Others have suggested that we simply live in so-called "privileged time" in which the special conditions that are benevolent for the evolution of biological life exist. But was time created at the moment of the big bang? Was there a biological precursor that initiated the big bang? Deep waters, these!
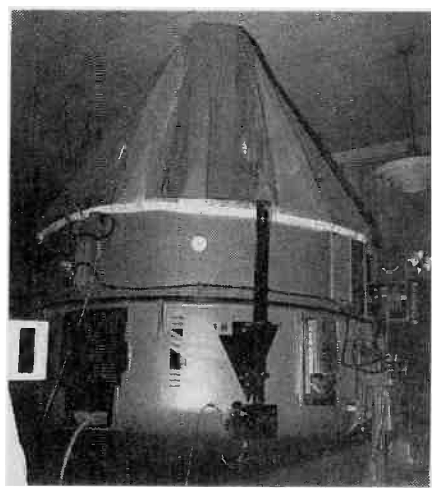
This is a biosensor assembly (used from 1973 to 1974) combined with skirted radio-telescope for detection of electromagnetic interference. A dust shroud protects the internal parts.
Who and Why? Today we must wonder if such transmissions over vast astronomical distances are the preferred mode of communication between advanced cultures in space. Of the four main classes of civilization, there are two types that could have acquired such an advanced technology: The "type-1 civilization", as it is called, would also be capable of interstellar and intergalactic travel, be immortal, and have missionary objectives. The "type-2 civilization" would only be capable of interstellar travel using fusion power with the gravity problem solved. Such beings would be regarded as "Gods of renown" by underdeveloped cultures.
The other two basic types reflect our own status quo: The "type-3 civilization" has electricity, basic creature comforts, is close to having fusion power, performs marginal interplanetary travel, but also has polluting industries, no gravity technology, and cancer. The "type-4 civilization" is primitive. It has basic tools, and fire, but no written language. Eidetic imagery dominates the spiritual life of such peoples.
Thus, then, only another type-3 civilization would have a technology similar to our own. Type-3 radio astronomers would know the precession frequency of neutral hydrogen (1.42 GHz) and frequency of the hydrogen radical (1.662 GHz). They would believe that interstellar communication is conducted at these universal constants because that's the way we understand it.
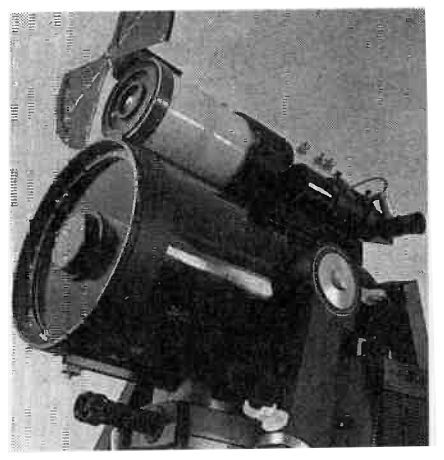
This is an electronically-controlled search telescope with top-mounted biosensor assembly. The Faraday tube is shuttered to prevent electromagnetic interference.
Unfortunately, throughout its 58-year-long history, radio astronomy has failed to detect one single, non-Gaussian signal from space. That's not surprising since truly advanced type-1 or type-2 civilizations are likely to have transcended electromagnetic communication altogether and would not step backwards just to accommodate scientifically underdeveloped cultures.
And so, if all of this is taken together, a totally new universe of technical possibilities unfolds before us. That biology holds the key to those communications should come as no surprise.
However, no matter what you might believe, biosensing is an exciting field. The secret ingredient is time. The deep-space image searches require great patience and the equipment must be frequently updated to suit arising technical and environmental situations. Emphasis is placed on electrophysiological phenomenon and technology, which come from the medical sector. The references provided in the boxed text should aid you in your own study of the field.
Literature and Patents.
- "Enzyme based methanol sensor", by W.J. Aston, R.E. Ashby, I.J. Higgins, L.D.L. Scott, and A.P.F. Turner, in Charge and Field Effects in Biosystems, Abacus Press, Turnbridge, UK, 1984, pp. 491-498. <http://amzn.to/1NuFMbT>
- Electrophysiological Methods in Biological Research, by J. Bures, Academic Press, N.Y., 1967. <http://amzn.to/1h7gHpU>
- Organic Semiconductors, by F Gutmann and L.E. Lyons, Wiley, N.Y., 1967. <http://amzn.to/1h7gKlA>
- "Biosensors," by C.R. Lowe, Trends in Biotechnology, Elsevier, Amsterdam, 2:3, 1984, pp. 59-65. <doi:10.1016/0167-7799(84)90011-8>
- Biosensors: Fundamentals and Applications, by A.F.P Turner, Oxford Univ. Press, Oxford, UK, 1987. <http://amzn.to/1h7gVgO>
- Sensor Having Piezoelectric Crystal for Microgravimetric Immunoassays, U. S. Patent 4,735,906, G.J. Bastiaans, April 5, 1988. <https://patents.google.com/patent/US4735906A/en>
- Immunoassay For Antigens, U. S. Patent 4,242,096, Oliveira, R.J. and S.F. Silver, December 30, 1980. <https://patents.google.com/patent/US4242096A/en>
Originally published in "Popular Electronics" (April 1991). Browse AmericanRadioHistory.com for complete issues of P.E. and many other historical radio and electrical publications in PDF format.
Return to the BIO-ICOMM Project homepage or the Bibliography of L. George Lawrence.